Scientists are investigating evidence of ancient terrestrial microbes preserved in Australia as well as mineral maps derived from Mars orbiter data to shed light on how to search for life on Mars.
Source: EOS.org
More than 3.5 billion years ago, two neighboring planets in our solar system probably resembled each other more closely than they do now. Both had running water and active volcanos. One planet was closer to the Sun, and tiny light-loving microbes evolved and flourished in rocky creches on that planet’s surface. When the microbes died, their bodies mixed with sand and sediment to form wavy layered mats that preserved the history of their early dominance in the planet’s geological record. Today similar microbes—and more developed creatures—still inhabit that planet, and we have even discovered the importance of those layered mats in our own origin story.
The second planet, farther from the Sun, provides more mysteries for us to ponder. We still don’t know whether our neighboring planet ever hosted life, though we have sent spacecraft to look for it. And using data from those missions, we have identified signs at various sites that liquid water—a necessity for life as we know it—flowed across the planet’s surface early in its history.
We’re about to become much better acquainted with one such site that, until now, has been known only from photos taken by satellites passing overhead. That’s because this location, where a lake once filled a crater called Jezero, is where the next Mars rover mission will land in February 2021. In a few weeks, the $2.42 billion Mars 2020 mission is scheduled to begin a 7-month voyage to Mars, where it will deliver the Perseverance rover to Jezero. Perseverance will explore the crater’s environs and collect samples intended to be returned to Earth by the NASA–European Space Agency Mars Sample Return mission, which is now starting to take shape.
Ancient History Recorded in Minerals
Jezero crater, named for a lakeside town in Bosnia and Herzegovina, was chosen as the landing site largely because it lies amid an ancient rock formation that contains the primitive mineral olivine (Figure 1) [Kremer et al., 2019]. On Earth, deposits of this greenish mineral provide important clues about the volcanic history of the regions where it is found, and Mars 2020 researchers hope that the Martian olivines will be equally informative.
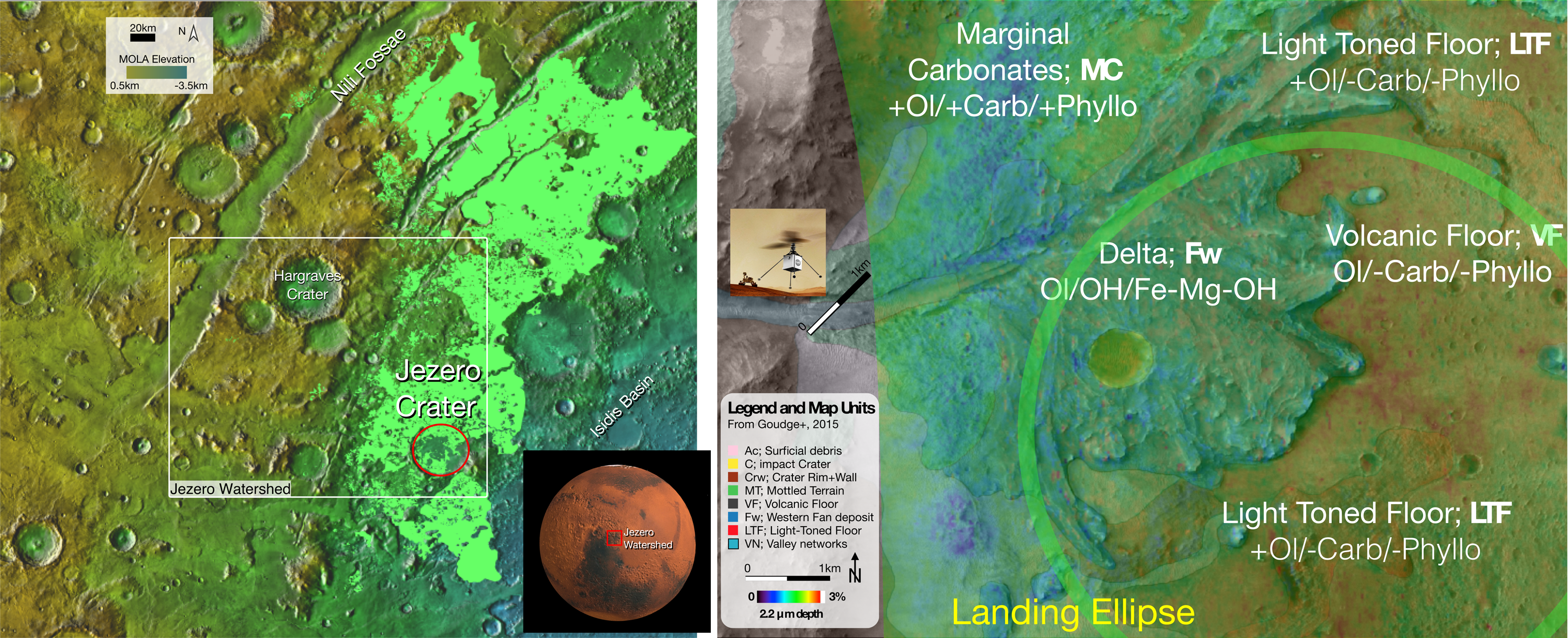
In 2003, researchers using data from the Thermal Emission Spectrometer on NASA’s Mars Global Surveyor spacecraft were the first to identify olivine at Nili Fossae (Latin for “Nile trenches”), a trough system near Jezero [Hoefen et al., 2003]. Later, the Thermal Emission Imaging System (THEMIS) aboard the Mars Odyssey orbiter was used to map this deposit further.
Excitement about the deposit then escalated when data from the Compact Reconnaissance Imaging Spectrometer for Mars (CRISM) aboard the Mars Reconnaissance Orbiter spacecraft—which has been helping earthbound researchers look for mineralogic evidence of past water on Mars since 2006—confirmed the presence of carbonate minerals associated with the olivine [Ehlmann et al., 2008]. Although olivine is associated with volcanism on Earth, carbonate minerals—often remnants of shells or bones—can be associated with life, though it’s too soon to say whether this holds true for Mars. This discovery shines new light on the formation and history of the olivine-carbonate rock within Jezero crater that the Perseverance rover will explore.In a recent study, we applied a new analysis technique to CRISM data and reported new details about an important rock unit in Nili Fossae. This rock unit, which has an outcrop in Jezero, is dominated by olivine and carbonates [Brown et al., 2020]. Our study also identified a region where no carbonates or clay units (formed from fine-grained sediments like river silt) are present, only olivine minerals with a large grain size, indicating that they formed by gradual cooling from a molten state. This discovery shines new light on the formation and history of the olivine-carbonate rock within Jezero crater that the Perseverance rover will explore.
Jezero crater once held a lake, fed by two inlet rivers, each of which deposited a delta of sediment at its valley mouth [Goudge et al., 2015]. Beneath the Jezero deltas is the olivine-carbonate unit, thought to have formed 3.82 billion years ago (Ga) on the basis of crater counting [Mandon et al., 2020], that the Mars 2020 mission will examine. This rock unit is incorporated into carbonate deposits around the edge of the crater that scientists recently hypothesized to have formed in standing lake waters [Horgan et al., 2020]. These scientists even suggested that stromatolites could be present in the “marginal carbonates” around the edge of the crater.
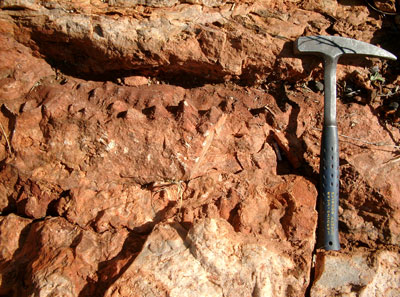
Here on Earth, stromatolites form when mats of photosynthetic microbes like algae trap sand and sediment, creating successive layers that can become lithified and preserved in the rock record (Figure 2). The Strelley Pool Chert, a deposit of dark, fine-grained silicate rock in the Pilbara region of Western Australia, is one of the few places on Earth where stromatolites of unequivocally ancient origin have been discovered. These 3.43-billion-year-old fossil microbes, which likely lived in a shallow marine environment [Allwood et al., 2006], constitute some of the best evidence of early life on Earth [Van Kranendonk et al., 2008], although single-celled life was already widespread on the planet by the time these stromatolites formed.
The similarity in age between the Strelley Pool stromatolites (3.43 Ga) and the olivine-carbonate lithology (3.82 Ga) in Jezero is striking. Could life on Mars have also gotten started quickly and taken advantage of a relatively clement surface environment on Mars before the planet was purged of its liquid water? Is there more we can learn about the first environments of terrestrial life that would inform our search for life on Mars?
Drawing Parallels Between Earth and Mars
A decade ago, we suggested that there was considerable similarity between the spectra of the olivine-carbonate lithology on Mars and of an ultramafic dunite (a type of magnesium-rich igneous rock) in the Pilbara in Western Australia [Brown et al., 2010]. The dunite is part of the 3.47-billion-year-old Mount Ada Basalt, a layer at the bottom of a rock sequence that is capped by the stromatolites in the 3.43-billion-year-old Strelley Pool Chert.
We noted that one way to simultaneously form phyllosilicates (clays) and carbonates in association with olivine is through a process called hydrothermal serpentinization. We posited that the flaky phyllosilicate minerals in the rock on Mars might be talc, in analogy with terrestrial talc-carbonate dunites from Earth’s Archean eon (4 to 2.5 billion years ago) [Viviano et al., 2013]. In particular, Archean dunite from Australia’s Mount Ada Basalt is an example of a magnesium-rich volcanic rock called komatiite, which has been suggested as a good analogue for Martian lava flows (Figure 3).
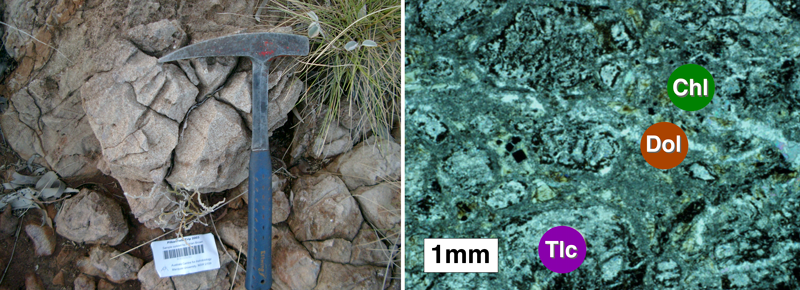
Komatiite forms when lava extrudes onto the surface, where it flows turbulently. Not just any kind of lava will form these rocks, however. It must be hot (roughly 1,400°C–1,600°C), have low viscosity (0.1–1 pascal second), come from Earth’s mantle, and be low in silica but high in magnesium and iron oxides (ultramafic). Komatiites are found almost exclusively in Archean shield areas like the Pilbara, which formed when Earth’s mantle was hotter than it is now.
Komatiite lavas are similar to Martian rocks with respect to geochemistry, morphology, and microtexture, and the presence of large-grained olivine crystals is a typical (although not unique) signature of komatiite lava. Can these rocks show us anything else about the physical properties of the Martian olivine-carbonate layer?
New Tools, New Findings, New Questions
In our recent study of the olivine-carbonate lithology observed at Nili Fossae, we used the 1-micrometer spectral band from CRISM data, which offers information on mineral composition and grain size, to produce mineral maps in three key regions of Nili Fossae [Brown et al., 2020]. We found that the olivine grains had to be between 500 micrometers and 1 millimeter across. If we assume the 1-millimeter grain size, the best fit to the data is an olivine with a moderate magnesium content, rather than the high magnesium content that had previously been suggested [e.g., Edwards and Ehlmann, 2015]. Perseverance should be well placed to determine whether the carbon dioxide source of the carbonates is volcanic or pyroclastic by looking for signs of rock fragments ejected from volcanoes.In our maps, the region displaying olivine 1-micrometer spectral bands with the greatest redshift (bands shifted to longer wavelengths) was located north of Jezero crater. We are still determining the relative contributions of compositional and grain size effects to this redshift [see Brown et al., 2020]. Interestingly, where we observed these most redshifted olivine spectra, we did not see spectral bands from carbonates or phyllosilicate. Assuming nearby carbonate and phyllosilicate minerals formed after the older olivine was weathered by the environment, our suggestion is that the olivine-only region could be a least altered “population-zero” part of the lithology.
Our study showed where carbonates are not being formed, but where did all the surrounding carbonate come from? Some studies propose that the early Martian atmosphere, which was much thicker than it is today and had higher carbon dioxide content, reacted with the olivine unit to form the carbonate. If this were the case, we would expect to see carbonate in the “basement unit” underlying the olivine; this is because this basement unit likely would have been exposed to the atmosphere at the same time as the olivine unit. There is no evidence for carbonate in the basement, however.
Instead, we entertained a suggestion that the carbonate may have been sourced from the Martian mantle at a time when carbon dioxide was more abundant in the mantle [Grott et al., 2011]. Perseverance should be well placed to determine whether the carbon dioxide source of the carbonates is volcanic (flowed along the ground) or pyroclastic (from an ash cloud) by looking for signs of rock fragments ejected from volcanoes. If Perseverance is then able to collect samples of the 3.82-billion-year-old olivine-carbonate lithology for eventual analysis on Earth, we may be able to better understand the history of carbon dioxide in Mars’s mantle using isotopic laboratory methods and to determine the provenance and alteration history of the carbonate we see near Jezero today.
The Thermal Inertia Problem
The THEMIS orbital instrument has mapped the entire Martian surface’s response to heat, providing evidence of what kinds of rocks are on the surface. Fine-grained materials like sand show a quick temperature rise in response to heating, but the temperatures of large rocks or lava flows on the surface respond more slowly.
The thermal response of the Martian olivine-carbonate lithology indicates it is composed of fine-grained materials [Rogers et al., 2018], evidence interpreted as being potentially at odds with the idea of the unit being a lava flow [Kremer et al., 2019; Mandon et al., 2020].
In our work, we addressed whether there is a correlation between the thermal inertia (a measure of a material’s response to heat) and the grain size of the olivine crystals we mapped using CRISM. We did not find any reliable correlation, however, complicating interpretations of the Martian olivine based on THEMIS data. We proposed this as “the thermal inertia problem” and suggested a couple of reasons why this apparent inconsistency exists based on our study of the Pilbara serpentinized komatiites. Today, these rocks, which originated as lavas long ago, are extremely friable and have a distinctive weathering style (Figure 3, left), but the mineral grains in them display sizes similar to the 500-micrometer sizes inferred for the Martian olivines (Figure 3, right). This observation suggests an explanation for the thermal inertia problem and perhaps offers more evidence supporting the idea that serpentinization occurred in the olivine unit on Mars. The Perseverance rover will have to study the problem in situ for us to answer these questions.
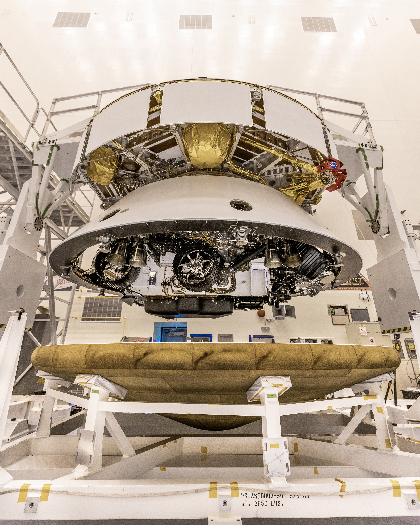
Countdown to Launch
The rover has been delivered to Cape Canaveral in preparation for a 22 July launch on an Atlas V rocket, and it will land at Jezero crater on 18 February 2021. Like the Curiosity rover before it, Perseverance will put earthbound observers through “7 minutes of terror” during its descent into the Martian atmosphere. If the landing is successful, Perseverance will be off to find the nearest olivine-carbonate outcrop or exposure.
Thanks to recent THEMIS and CRISM remote sensing studies and many prior studies, the Mars planetary science community is better prepared than ever to land at a geologically diverse and compelling landing site amid the most ancient rocks exposed on the surface. This landing site will allow us all to be mentally transported back in time to when the Jezero delta system was developing and liquid water was running on the Martian surface.
It was a different time, indeed, but we hope that the rocky microbial creche preserved in the ancient Pilbara can provide key insights for comprehending the ancient mystery of whether life ever developed on Mars.
Acknowledgments
This work was supported by the NASA Astrobiology Institute (grant NNX15BB01A) and the NASA Mars Data Analysis Program (grant NNX16AJ48G). T.A.G. acknowledges support from the CRISM team through a subcontract from the Johns Hopkins University Applied Physics Lab.
Source: EOS.org
Leave a Comment
You must be logged in to post a comment.